Operational Energy
Operational energy (OE) is the energy needed to run buildings. OE includes space climatisation, cooking, hot water, lighting and other appliances. In low income contexts it is very important because it requires a cash flow. Issues of embodied energy (EE) in the building materials are normally a much lower priority, unless direct savings are possible; even though in low energy buildings the lifetime EE may equal or even exceed OE.
In some developing countries cooking forms the largest home energy demand. Given increasing amenities, especially in the rapidly urbanising low- to middle income sectors, space cooling forms the main growth in energy demand. It can to a large extent be ensured by good building design using passive means including solar protection and natural ventilation – less easily in hot-humid climates, and not in polluted cities where outdoor air is undesirable. Being free, passive solutions are a priority. Small-scale air conditioning is energy intensive, not very efficient, and worsens the urban heat island effect. ELITH addresses housing, not the household technologies; our focus is on efficient and low-energy solutions both for individual buildings and, importantly, at the neighbourhood and urban scale, since some solutions are both more effective and cheaper at large scale. The research posits three main conclusions:
- Many solutions exist already, both traditional ones and ones resulting from modern research; but they are seldom implemented. Future efforts should focus far more on dissemination of existing best practice.
- The importance of embodied energy is as yet little understood in many developing countries, and should be focused strongly.
- For expanding hot climate cities there needs to be a drive towards urban scale solutions for cooling. This field is at present little known and even less practised.
At the scale of individual buildings, ELITH iincludes studies of regional housing types, energy use and trends in the partner countries, revised low-income house types, solutions for enhanced natural ventilation and passive cooling, and design options such as semi-outdoor cooking areas. Experience indicates that in both hot-dry and hot-humid climates, OE for space cooling can be reduced by 20-50% by good design, before the stage of adding technology. Research at urban scale has included studies of urban typologies in Asia and elsewhere regarding microclimate, thermal performance and socio-economic characteristics; comparative studies of urban residential blocks including a concept layout for improved microclimate and reduced energy needs (China); and investigation of large-scale district energy systems, in particular district cooling (DC). At urban scale too OE can be very considerably reduced by energy efficient design solutions.
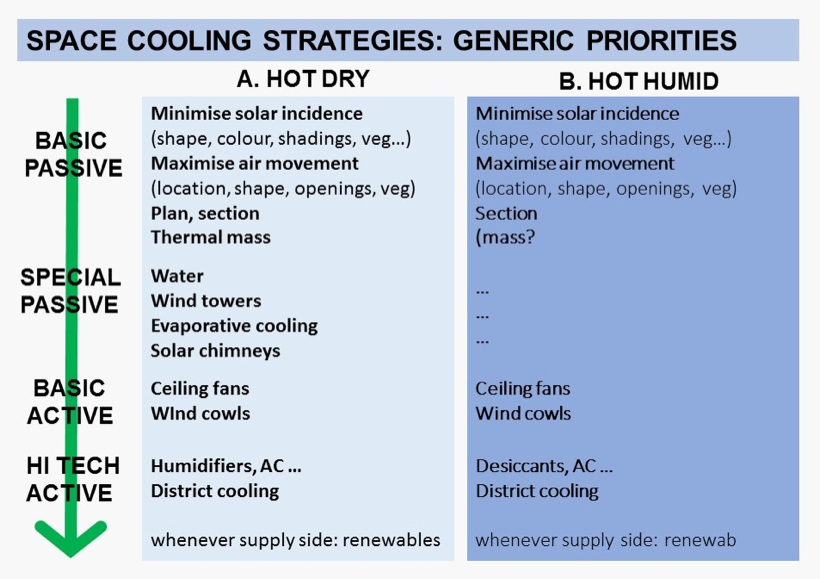
MORE about OE/OC and EE/EC
The fields of operational and embodied energy (or carbon) are quite well established but complex. Methodologies, background assumptions, system boundaries and contexts all require careful handling. The most common approach is LCA (life cycle analysis) which also has variants – adopted boundaries may be cradle-to-gate, cradle-to-grave or cradle-to-cradle – as well as limitations; for example the post-use phase does not account satisfactorily for residual energy/carbon. The focus of such studies also depends on the purpose; for example the perspective (and goals) of a manufacturer is different from that of a product designer, and different again from that of a climate scientist.
Within the field of buildings, and to a large extent for other products too, OE is most commonly accounted in terms of MJ or kWh, measured per area of building, to which one may add per year of lifetime or per whole assumed lifetime. EE is similarly accounted in terms of MJ per kg of building material or component, eventually aggregated as MJ per unit area of building. (normally unit of net or effective floor space).
For carbon, corresponding units are normally kg CO2e per unit area or per kg of product (sometimes per unit volume of product, for example per m3 of concrete). All other greenhouse gases being converted to CO2 equivalents; for example, 1kg of methane has the same greenhouse effect as about 21 kg of CO2.
One therefore arrives at an overall figure for a building’s performance which can be compared to other buildings, used to set up benchmarks or, in the design stage, to evaluate initial concepts and develop more favourable options.
Some particular issues:
Building lifetime is often assumed to be 50 years, which in our opinion is too short to be called “sustainable”. The lifetime assumption obviously has a big impact on the result.
Carbon is a key issue, due to climate change, and so carbon saved now is more “valuable” than carbon saved in say 100 years’ time, by which point one assumes the climate issue will have been solved (or else it’s too late anyway!)
Energy is a permanent issue, due both to costs and environmental impacts, but particularly today due to its correlation with climate emissions. This is only the case as long as most production is based on fossil fuels that generate those emissions. The energy footprint of buildings (and other products) will undoubtedly diminish over time, as production processes become more efficient and as energy supplies are more and more from renewable sources.
Embodied energy and carbon figures vary widely. For example, aluminium made using renewable hydropower (in Norway) requires the same production energy per kg but has a much lower embodied carbon impact than aluminium that is produced using oil or gas. If for example we use a database where the figures are based on ”EU average” for aluminium production, we cannot directly compare to a Chinese building where the aluminium is produced using energy from oil or coal. Similarly, in developing countries, factory efficiencies may be much lower those in, say, Europe. Hence, one must often revert to a detailed consideration of the primary energy picture.
Heat, work and power – thermodynamic energy quality – must be considered. For example, the energy of human labour, a large component of both costs and time in developing country rural construction, is almost negligible when compared to most energy inputs. For example, a piece of firewood weighing 1kg, grown for us for free by nature, contains the equivalent (in useful heat energy) of some 20-30 hours of hard human labour. The energy needed to construct a small timber house in Europe is around 100,000 kWh. This corresponds to roughly 300 years of human “work” – a telling reminder of how incredibly useful energy is to us. The actual human labour input into even a very labour-intensive rural African house is thus only a few per cent of the total EE; the labour energy in a modern building is only a fraction of one per cent of the total.
Energy or carbon? We refer here to energy throughout; the carbon (or climate) impacts of housing correspond broadly to those of energy, as long as energy supply systems are largely fossil-fuel based. A reduction of the carbon impact with significant decoupling occurs only if or when a country’s energy supplies are largely renewable. In the context of buildings, a major exception to this is the case of Portland cements, where the chemical process of production (calcination) emits more CO2 than the production energy, and is a large factor in the total lifetime footprint of many constructions. For sustainable buildings we must in addition consider toxicity to humans or the environment that may be neither energy nor climate related (including SO2, NOx, VOCs, formaldehyde, synthetic mineral fibres).
Operational versus embodied: until recently, in temperate climates such as Europe the energy used to heat buildings was by far the largest item. Today, with well insulated buildings the heat loss has been reduced to a fraction; and electrical appliances today including lighting are also far less energy consuming. The operational energy is thus being reduced to a much smaller part. So the embodied energy to produce the materials becomes a far larger part of the total; in advanced low energy buildings, the EE may in fact be half or more of the total impact even when calculated over the whole building lifetime. This is a picture that has only emerged over the last 15-20 years. It changes our understanding; and the part played by the materials assumes great importance. In so-called zero energy buildings (no net operational energy need at all, what little need there is being supplied by solar panels for example), to simplify somewhat one can say that the EE becomes virtually the only remaining component to consider.