PhD Research
At Warwick University my PhD research was within the Diamond Science and Technology group (named after the carbon-based material, not the Diamond Light Source!) in order to make better X-ray detectors for synchrotron beamlines.
I studied for my PhD at Warwick part-time, under the supervision of Prof. Mark Newton. I started my PhD in January 2016, and submitted my thesis in 2022.
PhD Thesis
My thesis, "Pixelated CVD diamond synchrotron radiation monitor with graphitic electrodes", is available on the University of Warwick publications service page, here.
Abstract
This Thesis describes the development and experimental testing of a new type of single-crystal chemical vapour deposition (CVD) diamond diagnostic instrument for synchrotron X-ray radiation. This pixelated detector is capable of non-destructively imaging the X-ray beam, and can remain in the beam path for the duration of synchrotron experiments. The detector can measure the position, profile, and flux of an incident beam. It has applications in beam position and profile monitoring, enabling active beam feedback and stabilisation.
The instrument utilises a single-crystal CVD diamond plate as the detector material, with laser-written conductive graphitic tracks embedded within the bulk diamond used as electrodes. The resulting instrument is an all-carbon X-ray imaging detector. Within the instrument's transmissive aperture there is no surface metallization that could absorb X-rays, and no surface structures that could be damaged by exposure to synchrotron X-ray beams. The all-carbon design ensures that there is no danger of electrode materials introducing new absorption edges that may affect synchrotron experiments.
A novel modulation lock-in readout scheme enables each pixel of the detector to be read out simultaneously. X-rays passing through the detector generate charge carriers within the bulk diamond through photoionisation, and these charge carriers travel to the nearest readout electrode under the influence of the modulated electrical bias. The signals from individual pixels are acquired by applying different modulation frequencies to different `bias' electrodes, and measuring the resulting signal amplitude of those frequencies on perpendicular `readout' electrodes. The system is designed to provide 100 frames per second image acquisition.
This instrument overcomes many of the issues associated with current generation synchrotron diagnostic instruments, enabling real-time and non-destructive X-ray beam profile measurements to be obtained.
Synchrotron Radiation Instrumentation
X-ray instrumentation plays a vital role in the work of scientists and users at Diamond Light Source and other synchrotrons worldwide. Synchrotron light sources are paticle accelerators used to produce beams of light and X-rays. These X-ray beams can be focused down onto sample just a few microns big, and are often billions of times brighter than even the most powerful hospital X-ray. A typical synchrotron looks like the picture below, courtesy of Wikimedia Commons.
Copyright © EPSIM 3D/JF Santarelli, Synchrotron Soleil
The ring is the synchrotron, a particle accelerator that circulates electrons at nearly the speed of light. The red shapes are magnets that bend the electron beam, causing it to emit synchrotron light, mostly X-rays. This light travels into the various beamlines shown branching out of the synchrotron. Each beamline contains different scientific instruments with which to perform experiments using the intense beam of X-rays that is produced.
As an example, shown below is the layout of one of these beamlines at Diamond Light Source. This is the B16 Test Beamline:
Synchrotron X-rays can be focused down to a micrometer in size to examine microscopic crystals, or to enable high resolution mapping of larger samples. These X-rays are used in scientific fields from archaeology to protein biology, and everything in-between. Common to all of the experiments conducted at synchrotrons is the requirement to maintain the stability of the X-ray beam on the sample over the whole range of timescales exploited by the synchrotron users, nanoseconds up to months!
It is of paramount importance to the users of synchrotron light sources that the X-ray beam remains stable, and pointint at the sample. Being able to accurately measure the intensity and the position of these intense, focused beams is extremely important.
Using diamond as an X-ray detector
The use of diamond as a detector of ionizing radiation is nothing new: it has been studied since the 1940s! Its exceptional mechanical, thermal, optical and electrical properties make it very well suited to the monitoring of highly intense beams of X-rays and other ionizing particles. The unique properties of single-crystal diamond make it an excellent choice for a “transparent” detector material for on-line synchrotron X-ray monitoring:
- high thermal conductivity and resistance to both thermal and radiation damage make it an ideal choice for insertion into intense X-ray beams;
- high carrier-mobility produces a detector with an extremely fast response to changes in the X-ray beam position or intensity;
- low atomic mass make it nearly transparent to X-ray light, whilst being just absorbing enough to detect the incident photons;
- and a (nearly) perfect diamond lattice produces very little X-ray scattering that could interfere with a user’s experiment.
The current state-of-the-art X-ray beam monitoring instruments are single-crystal diamond detectors, capable of measuring the synchrotron X-ray beam position with resolutions of a few 10s of nanometres, or better than 0.1% of the X-ray beam size, at kHz bandwidths. However, there are outstanding questions regarding how to best extract signal currents from the diamond, and how best to arrange surface electrodes to maximise the detector sensitivity to the incident beam motion and to measure the beam shape.
The high cost of the diamond has prevented its widespread use for all but the most extreme of applications, but single-crystal diamond, mostly dislocation-free and of exceptional purity is now available at a palatable cost. Innovations in CVD fabrication and polishing techniques mean that diamond windows a few millimeters in size and suitable for use as X-ray detectors can now be produced and used by the scientific community.
Synchrotron beamline instruments will typically use detectors made from diamond windows that are around 0.1mm thick, with electrodes deposited on opposite surfaces of the window and wire-bonded to a PCB frame or holder for the diamond. Standard lithography techniques allow the size and shape of the electrodes to be controlled: dots, quadrants, strips, and pixels can all be applied to the diamond surface.
Sufficiently energetic X-rays absorbed by the diamond will kick electrons from the valence band into the conduction band, forming electron-hole pairs. Under the influence of a bias voltage these charge-carriers travel to one of the electrodes where this current can be measured.
Some of these X-ray windows manufactured by different companies can be seen below, made by Dectris and Cividec Instrumentation. They both have four quadrants on the surface (with the wiring for these visible at the edge of the images), and this allows the position of the X-ray beam passing through the sensor to be found.
As the X-ray beam passes from one quadrant into the neighbouring quadrant the signals measured will change. This is what allows the X-ray beam position to be determined.
Innovative new techniques
My PhD focused on exploring new ways to improve upon the existing diamond detector technology.
The primary goal of my research was to produce an all-carbon detector, without the need for surface metallisation that A. unnecessarily absorbs part of the incident X-ray beam, and B. can easily be damaged by the intense X-ray beams. To do this, I utilised a novel method developed at the University of Oxford to laser-write arbitrary graphitic tracks within the bulk diamond. These tracks are conductive and can be used just as effectively as traditional surface electrodes but with the benefit of being safely buried under the surface of the diamond plate. The aim was to build upon the work carried out by Zhou et al who produced a pixellated diamond detector using delicate, traditional surface metallisation. The image below shows a sketch of (a) a standard, commerically-available four-quadrant X-ray beam detector; (b) the metallisation design of the pixellated detector produced by Zhou; and (c) the layout of the buried graphitic electodes used in my PhD research.
The secondary goal of my research was to develop a novel readout method to read all pixels simultaneously. The novel readout scheme developed for this detector utilises a different modulation frequency applied to each bias electrode, and then the individual modulation frequencies are detectable at the measurement electrodes. In the sketch below, the top figure shows a time-domain view of the modulation scheme. The bottom figure depicts a frequency-domain picture, showing how the individual modulation frequencies measured by the electrometer build up a picture of the beam profile upon the detector.
The detector resulting from this PhD was shown to work extremely well. It was tested at both Diamond Light Source (Oxfordshire, UK) and at European XFEL (Hamburg, Germany).
It is capable of transmissively imaging an X-ray beam profile. The X-ray absorption of the detector is an order of magnitude less than an equivalent fluorescent screen. A modulation lock-in readout technique has been demonstrated, enabling all pixels to be read simultaneously, and beam profile images to be obtained at 100 FPS with a 10 ms acquisition period.
Beam motions much smaller than the ‘pixel size’ can be easily resolved by applying 2D Gaussian fitting to determine the centroid, and the repeatability of the position measurement is better than 1 μm for 10 ms acquisitions. The pixel size could be varied by changing the spacing between the laser-written wire-electrodes during the fabrication process.
A measurement of individual XFEL pulses arriving at MHz rates has been carried out. Proof-of-principle XFEL X-ray pulse intensity, 1-dimensional spatial profile, and position measurements have been obtained.
The graphitic tracks survived exposure to both synchrotron and XFEL beam, and suffered from no noticeable radiation damage.
Background | Outreach and communication | PhD research | Publications |
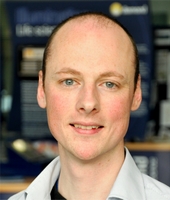
Chris Bloomer
c dot bloomer at warwick dot ac dot uk
Diamond Science &Technology Centre
University of Warwick
Gibbet Hill Road
Coventry
CV4 7AL
UK
chris dot bloomer at diamond dot ac dot uk
Diamond Light Source Ltd
Diamond House
Harwell Science &Innovation Campus
Didcot
OX11 0DE
UK