iMR CDT Research
Southampton: Cryogenic Magic Angle Spinning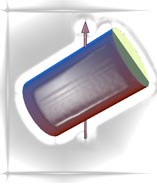
Magic angle spinning NMR at cryogenic temperatures: We are one of the few laboratories in the world with the capability of obtaining high-resolution NMR spectra of materials in the cryogenic temperature regime (below 77 Kelvin). Hyperpolarized singlet NMR: We are using DNP techniques to enhance nuclear polarization by many orders of magnitude and are developing techniques which use long-lived nuclear singlet states to maintain that polarization for long times; application to magnetic resonance imaging (MRI) is under development. Computational spin dynamics: We have developed algorithms to simulate the behaviour of many coupled nuclei, and are using advanced computer algebra to visualise and analyze magnetic resonance experiments.
St Andrews: High-Power High-Field, Pulsed EPR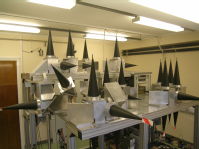
The vast majority of pulse EPR instrumentation in use today operates at 9.5 GHz at magnetic field strengths of 0.34 T. However there are substantial advantages in operating at higher frequencies and higher magnetic fields. The aim of the HIPER project at the Universities of St Andrews and Dundee was to construct a high field, low deadtime, pulse EPR spectrometer at 94 GHz operating at power levels several thousand times higher than equivalent commercial systems, with very high time resolution and instantaneous bandwidth and significantly increased concentration sensitivity. This required the development of advanced switches, isolators, antennas, fast pulse electronics and optics - many of which significantly advanced the state of the art. Today this system operates with state of the art performance and is primarily used in site-directed spin labelling and PELDOR studies for measuring long range distance and orientation distributions in large bimolecular systems. It is also used in conjunction with the the development of new Dynamic Nuclear Polarisation methodologies.
Nottingham: Dynamic Nuclear Polarization 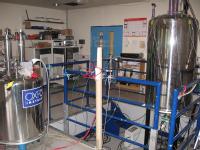
The research group led by Walter Köckenberger at the Sir Peter Mansfield Magnetic Resonance Centre of the University of Nottingham focuses on the use of various strategies to generate spin ensembles with very high spin polarisation. A topic of particular interest is the development of appropriate hardware to optimise the use of dynamics nuclear polarisation (DNP) in NMR spectroscopy and imaging. The group has designed and constructed a novel prototype spectrometer based on an unique dual centre magnet to combine low temperature DNP with liquid state NMR. Further hardware development currently aims at combining DNP with more sophisticated strategies for the implementation of fast temperature jumps such as laser induced melting. Another important focus of the group is the development of a theoretical framework to understand the complex spin dynamics of DNP experiments. In this project the group has made progress to simulate the spin dynamics of spin ensembles consisting of up to 104 coupled spins. Furthermore, the group has also an interest in parahydrogen induced polarisation and the use of optimal control theory to convert spin states into detectable magnetisation.
Aberdeen: Fast Field-Cycling MRI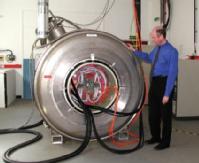
In standard MRI the magnetic field is normally held absolutely stable, to avoid image artefacts. However, this means that an MR system can only make measurements of the sample's (or patient's) NMR relaxation times at a single magnetic field. We are developing new types of MRI system, called “Fast Field-Cycling MRI” or FFC-MRI, in which the magnetic field can be switched (or cycled) rapidly between different values during the collection of an image, always returning to a fixed value to read out the signals that are used for image reconstruction. In this way extremely valuable information can be obtained about the precise way in which the relaxation times change as a function of magnetic field strength. We have already shown that this “T1-dispersion” information can monitor the process of blood clot formation (thrombosis) and that it can detect changes in cartilage in human joints arising from osteoarthritis. Our current research is aimed at improving the efficiency of field-cycling MRI, exploring interactions that could bring still more image contrast, and investigating bio-medical applications of the methods.
Warwick: NMR, DNP and EPR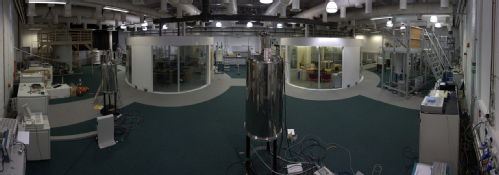
Our lab holds more than 12 spectrometers for EPR, NMR and DNP at magnetic fields up to 20 T. Our EPR research with diamond and silicon reveals the usefulness of these crystals for everything from water quality sensors to quantum computing. Solid-state NMR is a powerful tool for studying everything from batteries and fuel cells to pharmaceuticals. We apply this technique at high magnetic fields, and by simultaneously doing EPR also we can enhance the NMR sensitivity using dynamic nuclear polarization (DNP).
Dundee: Materials Physics EMR
Our focus is electron magnetic resonance (EMR) applied to materials physics, but as part of our wider St Andrews-Dundee collaborative centre in magnetic resonance we are also active in supporting applications of EMR to biochemistry and medicine. Core strength is in single crystal electron paramagnetic resonance (EPR) studies, aimed and detailed understanding of technological relevant paramagnetic centres in materials, and more recently we have extended this expertise to pulsed electron nuclear double resonance (ENDOR) providing further direct information of the local environment. We have an established track record in applying these methods to the study of point defects, including impurity ion defects, in ferroelectric and ferroic oxides. These typically have a perovskite related structure, and there is increasing interest in the development of novel multifunctional electronic device perovskite oxide heterostructures. We also have interests in energy materials, in particular materials for photovoltaic technologies; these include organic semiconductors, silicon, and II-VI and related semiconductors. We have expertise in electrically detected magnetic resonance (EDMR), these measurements provide direct information on the paramagnetic centres participating in spin-dependent transport within materials and device structures. The method has great potential to only in providing unique characterisation of electrically active centres, but also as a technological route of probing spin states. We perform both continuous wave and pulsed EDMR. Advances in the application of EMR methods to materials physics is enabled by innovation in instrumentation and techniques. We are currently gaining a more complete understanding of EDMR instrumentation and techniques aimed at overcoming some of the practical difficulties often encountered in performing these experiments, for example amplifier configuration, suitability, and design. We are also interested in developing EMR techniques for probing interactions and correlations between local (polarisations), and external, electric fields and paramagnetic centres, and in collaboration with Graham Smith we have a core interest in advancing high frequency EMR instrumentation for the detailed materials and nanoscale device characterisation.