Multiscale Modeling of Mass Transport in Disordered Media: from Drug Delivery to Freezing Tolerance
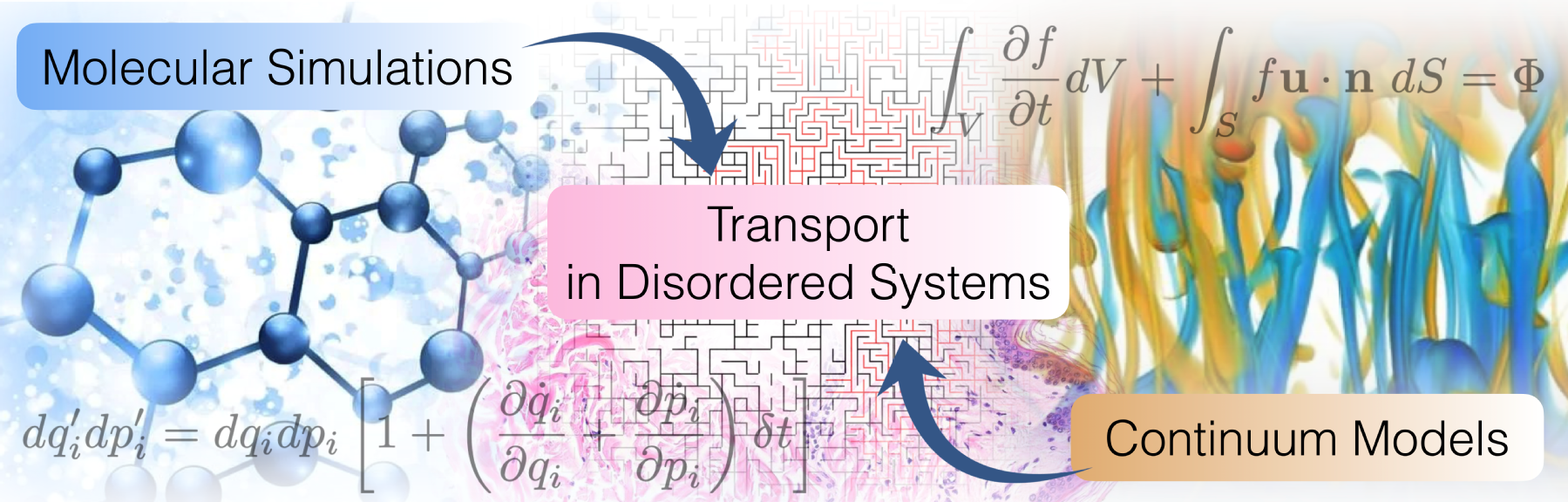
Supervisors: Gabriele Sosso and James Sprittles
Summary:
Understanding how molecules move through disordered networks is a fundamental question that has an impact on countless practical applications. For instance, many medicinal drugs are delivered through our skin - a very complex system. Another example is the percolation of ice through plant cells, which can massively reduce crop yield. Until now, the modelling of these applications has relied on either continuum fluid dynamics or molecular simulations. With this project, we will build a bridge between the two disciplines, thus developing an understanding of the relationship between (macroscopic) mass transport and the (microscopic) structure of biological disordered materials.
Background: This project originates from a recent attempt to understand the porosity of the cell wall [1], i.e., the biological tissue surrounding plant cells. In particular, we are interested in the percolation of ice through the cell wall, a process that has huge impact on the freezing tolerance of plants [2] – and on the quality of French wine [3] as well!
The current paradigm to assess the porosity of the cell wall consists in staining the cell wall with a fluorescent dye and measure the reduction in the fluorescent intensity of the system upon the insertion of a “quencher” molecule, i.e., a molecule that quenches the fluorescence of the dye [4]. Our experimental collaborators in Durham have observed that different, genetically modified cell walls are characterised by different quenching efficiencies, which in turn are proportional to the ease by which the quencher percolates through the cell wall. This is a rather indirect measure of the porosity of the tissue – but at the moment we cannot make a connection between these macroscopic observables and the microscopic structure of, in this case, the cell wall network, which we can imagine to be similar to a percolation grid [5] (Fig. 1c).
Similar issues emergence in a variety of other scientific fields. An example is transdermal drug delivery, which involves drug molecules percolating through the disordered network of the stratum corneum, the outermost layer of our skin [6]. The design and manufacturing of porous materials for sensing application is another example of great relevance for industrial applications [7]. In all this cases, we struggle to build a link between macroscopic, continuum models, and microscopic, molecular-level simulations [8].
Aim of the project: This project seeks to understand mass transport in disordered system, by bringing together the expertise of Prof. Sprittles (continuum models) and Dr. Sosso (molecular simulations). In particular, we are aiming to:
- Make a connection between experimental observables (such as the permeability of drug molecules) and the molecular structure of the biological media involved (such as the porosity of biological tissues).
- Design truly multiscale, continuum models for mass transport in disordered media, informed by microscopic insight from atomistic and/or coarse-grained molecular dynamics simulations.
- Apply the resulting computational framework to tackle specific applications of practical relevance, namely transdermal drug delivery, plant cells cryopreservation and gas sensors.
Methodology: We aim to realise a bottom-up approach based on the calculation of selected quantities such as the diffusivity of water and ice through the cell wall or the interaction strength between a given drug molecule and the constituents of the stratum corneum. These quantities will then be fed into continuum models, which we can validate with experimental data, thanks to academic (Prof. Heather Knight, Durham) and industrial (Cryologyx) collaborators as well. Crucial to the success of this project is the HetSys training program, which is unique in that it will provide the student with a rare blend of skills, from atomistic simulations to fluid dynamics.
It is important to stress that whilst we will focus on specific problems and applications – with the aim of maximise the impact of our research – our goal is to craft models and theoretical frameworks that would be applicable to a variety of different systems, thus furthering our understanding of the fundamental process of mass transport in disordered media.
Going beyond the state of the art: At the moment, there is a substantial gap between continuum models and molecular simulations, largely due to the different time and length scales involved. With this project, we are seeking to build a bridge between the two disciplines by leveraging the unique training program offered by the HetSys CDT – which encompasses fluid dynamics as much as atomistic simulations.
In addition, thanks to the (free of cost) support of collaborators at Warwick (Prof. Matt Gibson, Warwick Chemistry and WMS) and Durham (Prof. Heather Knight, Department of Biosciences), we are in the unique position of being able to build upon experimental data that we can use to validate our models directly. Examples include fluorescence microscopy data aimed at quantifying the mass transport through biological materials – data that Sosso and Sprittles have already started to look at.
Links to HetSys Training. Crucial to the success of this project is the ability of the PhD student to be able to work with different computational techniques encompassing very different time and length scales, from atomistic (PX911) to continuum (PX912) simulations. The HetSys training program is explicitly designed to provide this unique portfolio of skills. The collaboration between Chemistry and Mathematics at Warwick is the first proposed within the HetSys training scheme. This project has implications on drug delivery as well as ice percolation, with the former critical for healthcare technologies and the latter key in the context of plant biology; two disparate application foci.
References
- D. J. Cosgrove, “Growth of the plant cell wall,” Nat Rev Mol Cell Biol, vol. 6, no. 11, pp. 850–61, Nov. 2005, doi: 10.1038/nrm1746.
- Y. Ding, Y. Shi, and S. Yang, “Advances and challenges in uncovering cold tolerance regulatory mechanisms in plants,” New Phytol., vol. 222, no. 4, pp. 1690–1704, 2019, doi: 10.1111/nph.15696.
- J. Partridge, “French wine suffers worst hit in decades amid damage from frost and disease,” The Guardian, Sep. 07, 2021. Accessed: Oct. 12, 2022. [Online]. Available: https://www.theguardian.com/business/2021/sep/07/french-wine-output-frosts-weather
- X. Liu et al., “Novel tool to quantify cell wall porosity relates wall structure to cell growth and drug uptake,” J Cell Biol, vol. 218, no. 4, pp. 1408–1421, Apr. 2019, doi: 10.1083/jcb.201810121.
- A. G. Hunt and M. Sahimi, “Flow, Transport, and Reaction in Porous Media: Percolation Scaling, Critical-Path Analysis, and Effective Medium Approximation,” Rev. Geophys., vol. 55, no. 4, pp. 993–1078, 2017, doi: 10.1002/2017RG000558.
- T. R. Mollee and A. J. Bracken, “A Model of Solute Transport through Stratum Corneum Using Solute Capture and Release,” Bull. Math. Biol., vol. 69, no. 6, pp. 1887–1907, Aug. 2007, doi: 10.1007/s11538-007-9197-x.
- C. McDonagh, P. Bowe, K. Mongey, and B. D. MacCraith, “Characterisation of porosity and sensor response times of sol–gel-derived thin films for oxygen sensor applications,” J. Non-Cryst. Solids, vol. 306, no. 2, pp. 138–148, Aug. 2002, doi: 10.1016/S0022-3093(02)01154-7.
- A. Zech and M. de Winter, “A Probabilistic Formulation of the Diffusion Coefficient in Porous Media as Function of Porosity,” Transp. Porous Media, Jan. 2022, doi: 10.1007/s11242-021-01737-5.
Are you interested in applying for this project? Head over to our Study with Us page for information on the application process, and the HetSys training programme.
For the 2023/24 academic year, UK Research and Innovation (UKRI) funding is open to both UK and International research students. Awards pay a stipend to cover maintenance as well as paying the university fees and providing a research training support grant. For further details, please visit the HetSys Funding Page
At the University of Warwick, we strongly value equity, diversity and inclusion, and HetSys will provide a healthy working environment dedicated to outstanding scientific guidance, mentorship and personal development. Read more about life in the HetSys CDT here.
HetSys is proud to be a part of the Physics Department which holds an Athena SWAN Silver award, a national initiative to promote gender equality for all staff and students. The Physics Department is also a Juno Champion, which is an award from the Institute of Physics to recognise our efforts to address the under-representation of women in university physics and to encourage better practice for both women and men.