DUNE
Academics: Prof Gary Barker, Dr Xianguo Lu, Dr John Marshall
Postdoctoral Fellows: Dr Maria Brigida Brunetti, Dr Andy Chappell
Students: Matthew Osbiston, Federico Battisti, Komninos-John Plows, Matthew Snape
Associate Members: Dr Marian Ivanov
The Deep Underground Neutrino Experiment (DUNE) is a future neutrino experiment in the USA. It will use the world’s most intense neutrino beam, and cutting-edge Liquid-Argon Time-Projection Chambers (LArTPC) detectors, to help answer some of the biggest outstanding questions about the nature of our universe.
Overview
There are three known types of neutrino: electron, muon and tau. Neutrinos have the unusual property that they change from one type to another as they travel a few hundred km; this is known as "neutrino oscillation". DUNE will initially produce around 1.2 MW beams (with plans to increase it later) of muon neutrinos or muon antineutrinos at Fermilab in Chicago; the properties of this beam will be first be measured by a detector only 600 m from where the neutrinos are made, which is too short a distance for changes of type to occur. The beam will then travel 1300 km through the earth to a huge underground far detector at the Sanford Underground Research Facility (SURF) in South Dakota, which will measure it again to look for changes in neutrino type.
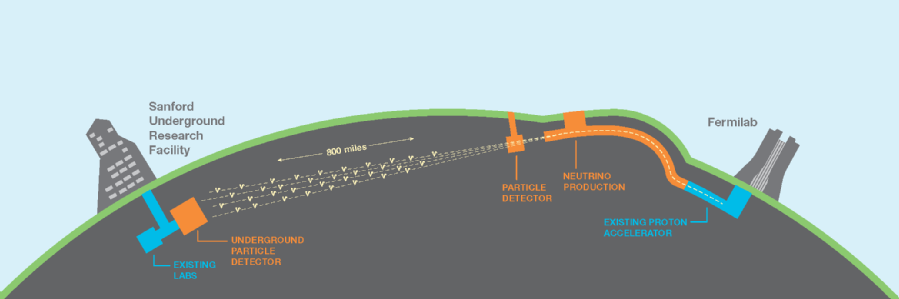
DUNE scientists will look for differences between neutrinos and antineutrinos in the way they change type. This could provide an answer to one of the most fundamental questions in physics: why do we see only matter in the Universe when equal quantities of matter and antimatter were created in the Big Bang? Neutrinos may hold the key to this mystery, and DUNE will enable scientists to find out.
The difference in neutrino oscillation between muon neutrinos and muon antineutrinos is quantified by a parameter called δCP, which can take any value from 0 to 360 degrees. If δCP is 0 or 180 degrees, there is no difference in neutrino oscillation between neutrinos and antineutrinos. The further away from 0 or 180 degrees the value of δCP, the larger the difference in oscillation between neutrinos and antineutrinos.
DUNE will make measurements of the value of δCP by looking at differences between oscillation from muon neutrinos to electron neutrinos and muon antineutrinos to electron antineutrinos. For this reason, the neutrino beam will be of muon neutrinos 50% of the time and muon antineutrinos the other 50%.
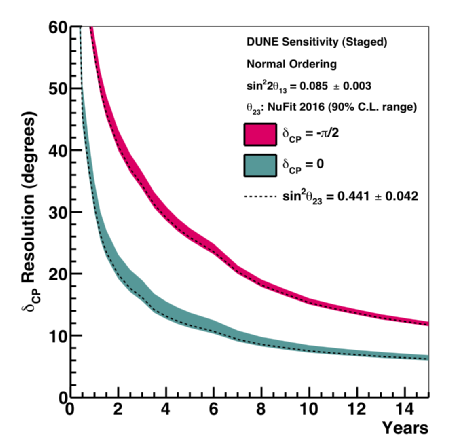
To make these measurements, it is essential to have a very large far detector. The DUNE far detector will consist of four large liquid argon time projection chambers (LArTPCs).
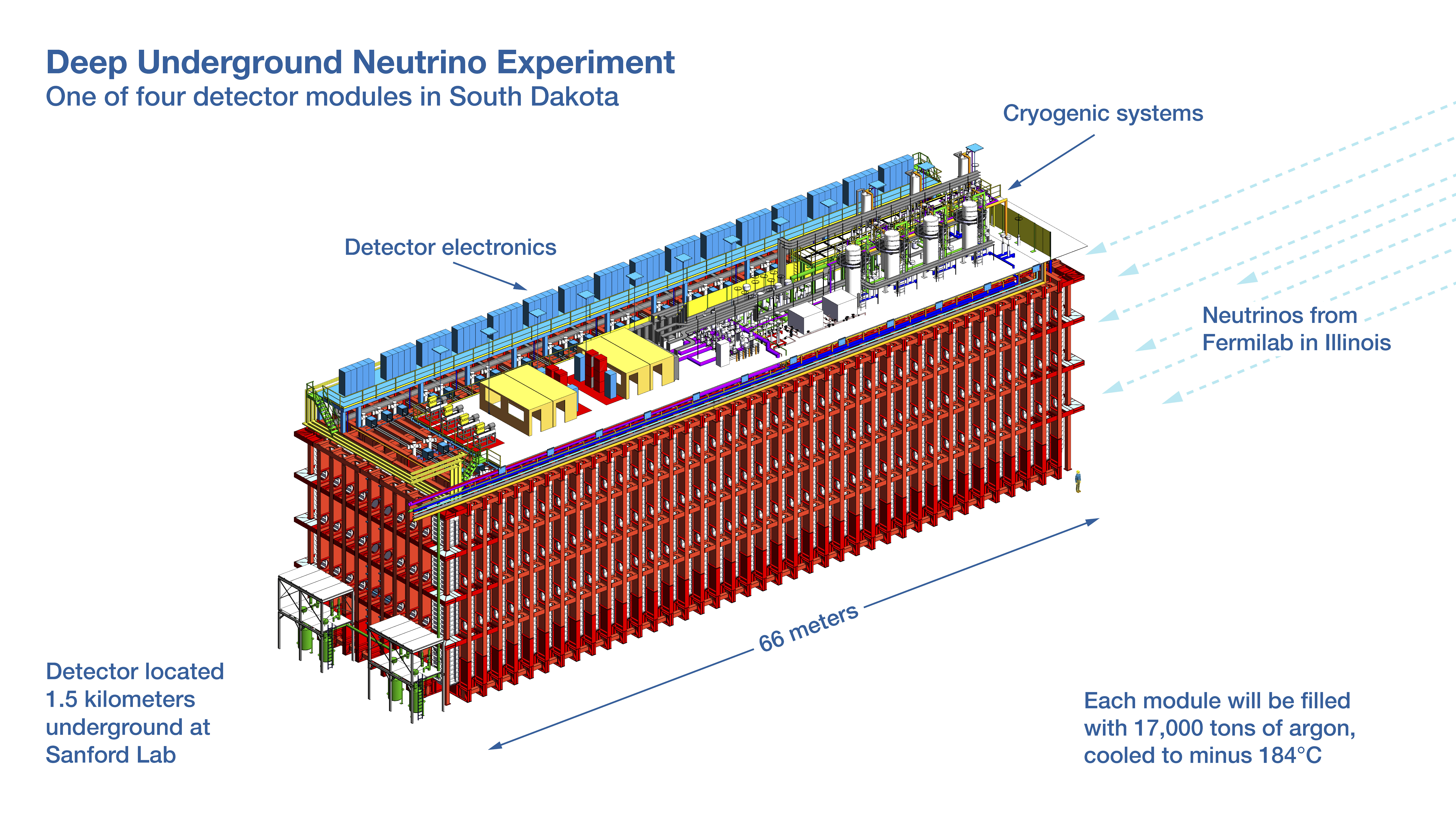
The LArTPCs will be located 1500 metres underground at SURF, which is in a disused gold mine in South Dakota. Each of the four detectors will contain 10000 metric tons of liquid argon cooled to -190oC. LArTPCs give excellent tracking of charged particles as they pass through the detector, and their energies and positions in the detector can be measured very accurately.
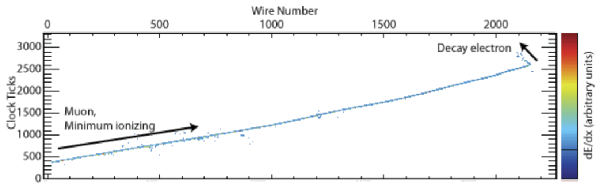
Other aims of DUNE are:
- Neutrino mass hierarchy: oscillations between one neutrino type and another take place according to the mathematics of quantum mechanics. This mathematics tells us that oscillations can only occur if neutrinos have different masses. To be precise, oscillations depend on the difference between the squares of the masses of two of the neutrinos. These squared masses are usually written as m12, m22 and m32. We know from observations of neutrinos from the Sun that m22 > m12, but it is not currently known whether m32 is > m12 and m22 or < m12 and m22. This ordering of the squared masses is known as the "neutrino mass hierarchy"; DUNE will make measurements that aim to determine which ordering is correct.
- Search for physics beyond the Standard Model including proton decay, heavy neutral leptons and non-standard neutrino interactions.
- The far detector will be ready to detect low-energy neutrinos from a core-collapse supernova if one occurs.
Physicists at the University of Warwick are making several important contributions to DUNE; please look here for more details about them.
Event Reconstruction
The use of LArTPC imaging detectors at DUNE is a paradigm shift in neutrino physics. The detectors are capable of producing "photograph-quality" images of the charged particles that emerge when a neutrino interacts. Such images can be extremely complex, with multiple overlapping particles, and particles that interact again or decay.
The sensitivity to physics at DUNE depends critically on the ability to interpret the details in the complex images. Physicists need to "reconstruct" the interactions, by identifying the types of particles emerging, their energies and their directions. The human eye/brain can be quite adept at analysing the images, but it's still a significant challenge for computer algorithms to accurately and robustly mine the images for the physics information they contain.
The Warwick EPP group is the centre of development for the Pandora LArTPC event reconstruction, which aims to bring a "multi-algorithm" approach to interpreting interactions recorded by LArTPC detectors. This approach is described in the Documentation repository hosted on the GitHub pages of the Pandora software project, here.
In essence, features in events are built up gradually, with separate algorithms to implement each incremental step. For each step, traditional clustering algorithms can be applied, machine-learning approaches can be used (including the latest "deep-learning" approaches), and physics and detector knowledge can be carefully built into the algorithms.
There is an important continuum between the later steps of the event reconstruction and the first steps of a physics analysis at DUNE, so a vital area of work is to optimise the event reconstruction for DUNE's physics programme.
A PhD project in Warwick would concentrate on an area of specific physics interest at DUNE; recently this has included the flagship neutrino oscillation analysis, examination of supernova neutrinos and nucleon decay. The reconstruction of events recorded by the LArTPC detectors could be optimised to meet the needs of the physics analysis, by identifying current image-analysis limitations and designing new algorithms to improve the pattern recognition. Work would then proceed to perform the analysis itself, including selection of events, parameter fitting and consideration of systematic uncertainties, all in conjunction with the relevant DUNE physics working group.
ProtoDUNE
The ProtoDUNE Single Phase (SP) detector is the prototype of the DUNE far detector modules located in the CERN North Area. ProtoDUNE-SP contains 770 tonnes of liquid argon (420 tonnes are in the active volume of the LArTPC). The installation of the ProtoDUNE-SP was completed in July 2018. The full data-taking started in October 2018.
One view of the ProtoDUNE-SP LArTPC with coordinates and major components labelled.
A charged particle test beam produced by the Super Proton Synchrotron (SPS) enters the LArTPC through a beam plug. This test beam simulates the charged particles resulting from neutrino interactions at DUNE FD in the closest way possible, with a momentum range from 0.3 to 7 GeV/c. The high-quality ProtoDUNE-SP data can be used for detector calibration and particle reconstruction. The test beam consists of protons, pions, muons, kaons and positrons so that one can do physics measurements on these charged particles and their interactions with the argon nucleus.
ProtoDUNE-SP data 1 GeV/c charged-pion candidates.
The DUNE Warwick group is analysing the data from ProtoDUNE. With the positively charged pion beams, we not only can demonstrate the state-of-the-art LAr technology but also aim to extract the intranuclear dynamics inside the argon nuclei that are crucial for the future neutrino oscillation programs at DUNE.
Detector Calibration and Particle Identification
The main physics goals of ProtoDUNE-SP are to perform the detector calibration and achieve robust particle identification (PID). The reconstructed particle energy loss per unit length (dE/dx) can be used as a calorimetric-based PID method. The particle dE/dx is measured by converting the charge collected by the wires to energy. Therefore, understanding the charge response of wires in a LArTPC is important, and it is essential to determine the energy scale and correct the non-uniformities in the detector response.
Detector Calibration
The main calibrations are:
- Calibration of space charge effect (SCE) due to the ion accumulation caused by the cosmic muons. The SCE will distort the electric field in a LArTPC and affect the reconstructed particle energies and trajectories.
- Calibration of drift electron lifetime caused by the impurity in Argon capture the ionized electrons. This effect will lower and bias the amount of charge measured on the wire planes.
- Calibration of the remaining non-uniformities in the detector response, such as charge calibration based on cosmic-ray muons.
Throughgoing cosmic-ray muons dQ/dx distribution before and after calibration.
Particle identification: protons and muons
Protons deposit a large amount of energy in the region at the end of their track, whereas pions behave as minimum ionizing particles (MIP) in the LArTPC. The particle dE/dx after calibration is plotted against its residual range. A chi-square test is performed with the assumption of a stopping proton. The value of this computed chi-square can indicate whether this particle is a proton-like or a pion-like candidate.
dE/dx versus residual range after calibration for the stopping protons (upper band) and the muons (lower band).
Near Detector
The role of the Near Detector (ND) of DUNE will be to operate as the experiment’s control. Located very close to the neutrino beam source (about 600m away), the ND will establish the experiment’s null hypothesis by measuring the un-oscillated neutrino flux; it will also provide constant monitoring of the status of the neutrino beam, constrain systematic uncertainties, and provide essential measurements of the neutrino interactions on Argon to improve models. The ND complex will include three primary detector components: a liquid argon TPC called ND-LAr, a high-pressure gas TPC called ND-GAr and an on-axis beam monitor called SAND. The three detectors will serve important individual and overlapping functions, with ND-LAr and ND-GAr also able to move transverse to the beam’s axis via the DUNE-PRISM program.
View of ND On-Axis (upper) and Off-Axis (lower). Detectors from left to right: SAND, ND-GAr, and ND-LAr.
The DUNE Warwick group is contributing to the development of state-of-the-art tracking algorithms as well as benchmarking the physics capabilities of ND-GAr. The detector’s main goal will be to study neutrino interaction kinematics, while also acting as a spectrometer for muons produced in ND-LAr. The gaseous argon used in ND-GAr’s TPC offers an ideal compromise between the necessity for a target which is massive enough to produce a substantial sample of neutrino interactions and a relatively low-density medium with lower tracking thresholds than liquid/solid materials (i.e. ND-LAr/SAND). Being capable of tracking lower energy particles, ND-GAr will act as a “magnifying glass” for nuclear effects in neutrino-on-argon interactions, which are currently poorly understood and majorly contribute to the systematic uncertainties in the neutrino oscillation measurements.
Schematic view of ND-GAr.
We are also working on generic R&D of a high-pressure TPC (WarTPC) with potential application for the DUNE ND-GAr, in particular on a new design that can allow optimal neutrino-hydrogen measurements [Phys. Rev. D 102, 033005 (2020)]. This hydrogen-rich high-pressure TPC R&D is part of the EU AIDAinnova Project (Task 7.4) and the overall R&D project also received support from the STFC Early Technology Development Capital Funding.