Some recent examples of our research
Plating Zn better: Electrolyte additives for preventing dendrite formation
Advanced Materials. 2023, 2307708
Preventing the growth of dendrites is a universal problem for metal electroplating, and is a fundamental limitation to implementing high capacity metal anodes. This remains true even for aqueous zinc batteries, and represents one of the main sources of capacity loss. Introducing electrolyte additives that cause 'electrostatic shielding' - that is, cations that preferentially buildup around dendrite growth sites - is a developed strategy in preventing dendrite growth for Li metal anodes. Here, PhD student Yi Yuan explores the role of this mechanism for the case of zinc metal anodes, using LiCl as an additive, and reveals the significant influence it has on the resulting electroplating morphology by operando electrochemical TEM imaging.
--
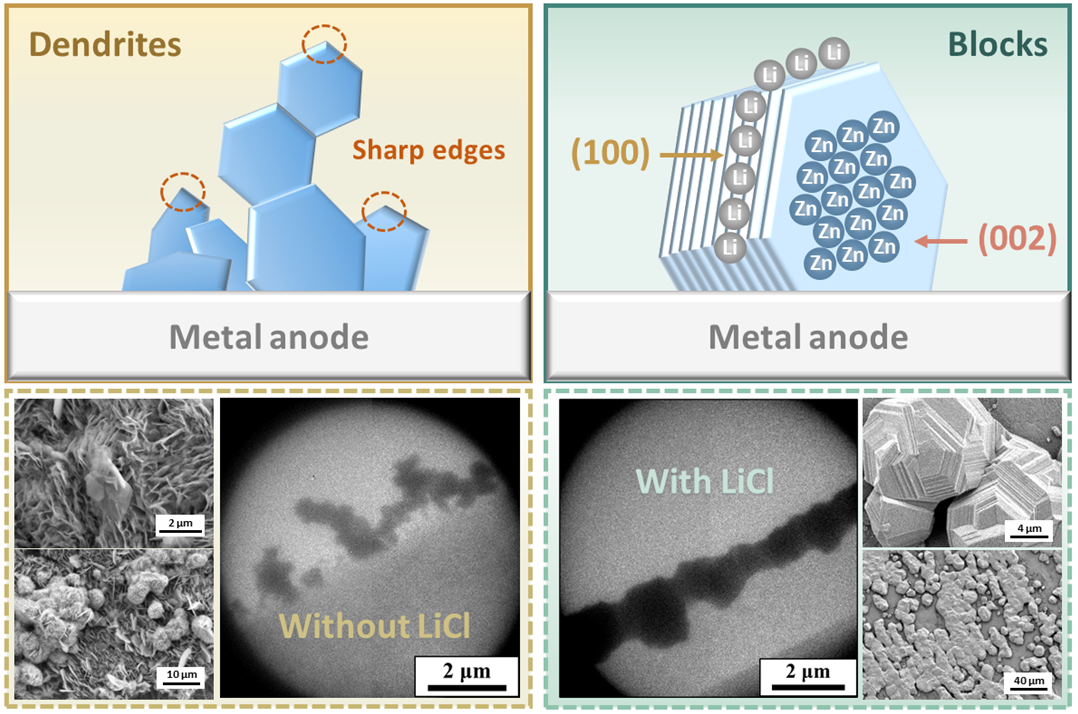
--
--
--
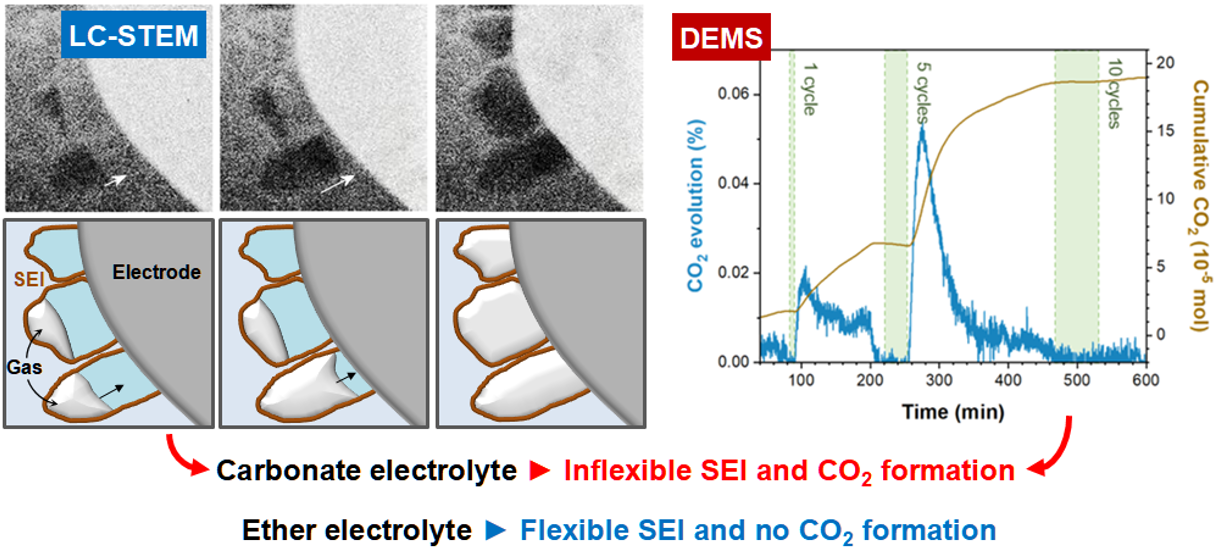
The importance of the solvent for sodium-ion battery electrolytes
Energy & Environmental Science. 2023, 16, 535-545Link opens in a new window
Sodium metal anodes are a part of the promise of cheap and high performance sodium–ion rechargeable batteries. However, they suffer from endemic low cycling performances. Perhaps the most powerful strategy to combat these failures is to use an appropriate electrolyte, one that will react and coat the sodium anode in a robust solid–electrolyte interphase (SEI) that is robust to degradation. Here, PhD student Chen Gong performed operando electrochemical transmission electron microscopy to image the sodium metal anode in carbonate and ether based electrolytes, revealing the role of gas evolution in causing poor performance with carbonate solvent electrolytes.
--
What causes capacity loss during ageing for metal anodes in aqueous zinc batteries?
Joule. 2023, 7, 366-379.Link opens in a new window
The search for batteries beyond Li-ion that offer better performance and safety has led researchers to explore the advantages of Zn-ion batteries. Zinc is relatively unreactive, raising the prospect of a rechargeable battery that can use a simple aqueous electrolyte; a cheaper, safer option to the organic electrolytes that must be paired with reactive lithium. However, water still reacts with the zinc in corrosion reactions. This consumes zinc, lowering the battery’s capacity, and generates gas that accumulates in the sealed cell. In this work, PhD student Shengda Pu, working with experts in X-ray computed tomography at Oxford, diagnoses the contribution of corrosion to performance decay in zinc batteries and reveal the critical role of gas accumulation in deactivating large sections of electrode, which cripples cell performance.
--
--
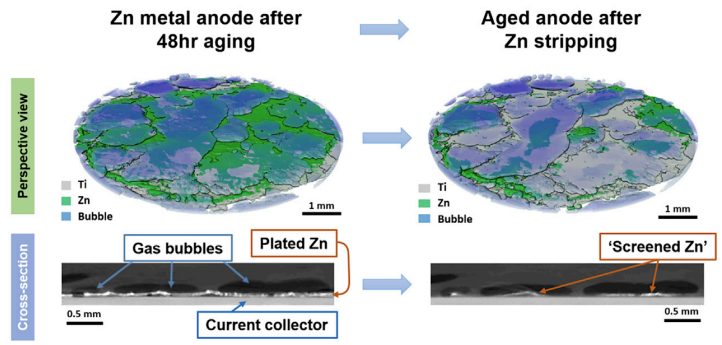
--

Identifying the importance of fluoride in making a good interphase for lithium anodes
Advanced Energy Materials. 2021, 11, 2003118.Link opens in a new window
Lithium metal is perhaps the perfect anode material for a high capacity rechargeable battery. Unfortunately, there is a reason we use graphite in current Li-ion batteries; lithium metal is highly reactive, leading to continual side-reactions with the electrolyte, and also prone to forming dendrites, which can detach and become electrically isolated. These mechanisms represent the main causes of capacity loss for Li anodes. Ideally, we would design the interphase layer - the solid electrolyte interphase (SEI) - that forms over Li when it reacts with the electrolyte so that it was robust to battery cycling and favoured smooth deposition and dissolution of the Li metal. Here we use a combination of liquid-cell TEM and mass spectrometry to explore how tailoring the interphase by inclusion of fluoride additive in the electrolyte changes the cycling behaviour of Li.
--
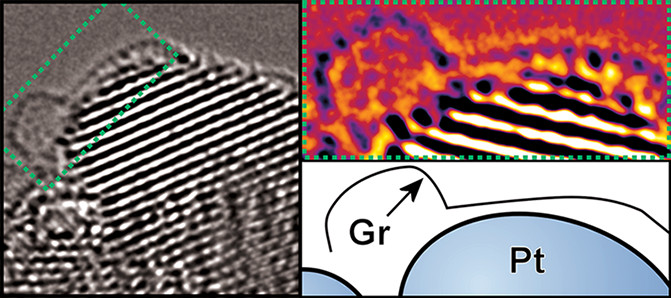
Designing 'nano-shields' to protect valuable platinum nanocatalysts
ACS Appl. Energy Mater. 2021, 4, 1286-1293.Link opens in a new window
Platinum is one of the most effective electrocatalyst materials, with Pt nanoparticles being the standard to beat for important reactions like the hydrogen evolution reaction, a reaction allowing us to make hydrogen from water with electricity, and the oxygen reduction reaction, a critical reaction for the functioning of hydrogen fuel cells. Platinum is expensive, representing a major bottleneck in commercial scale-up of hydrogen energy, and so we want to minimise its use. Part of this means addressing the loss the platinum undergoes during operation, which necessitates us pre-loading excess Pt to pre-compensate for this forecast loss. Here, building on our prior work in ACS Nano with collaborators at the Korea Institute of Energy Research (see cover, right), we developed a way of shielding the Pt catalyst with a bacteria-derived graphene shield. This allowed for the extended robust operation of the catalyst.
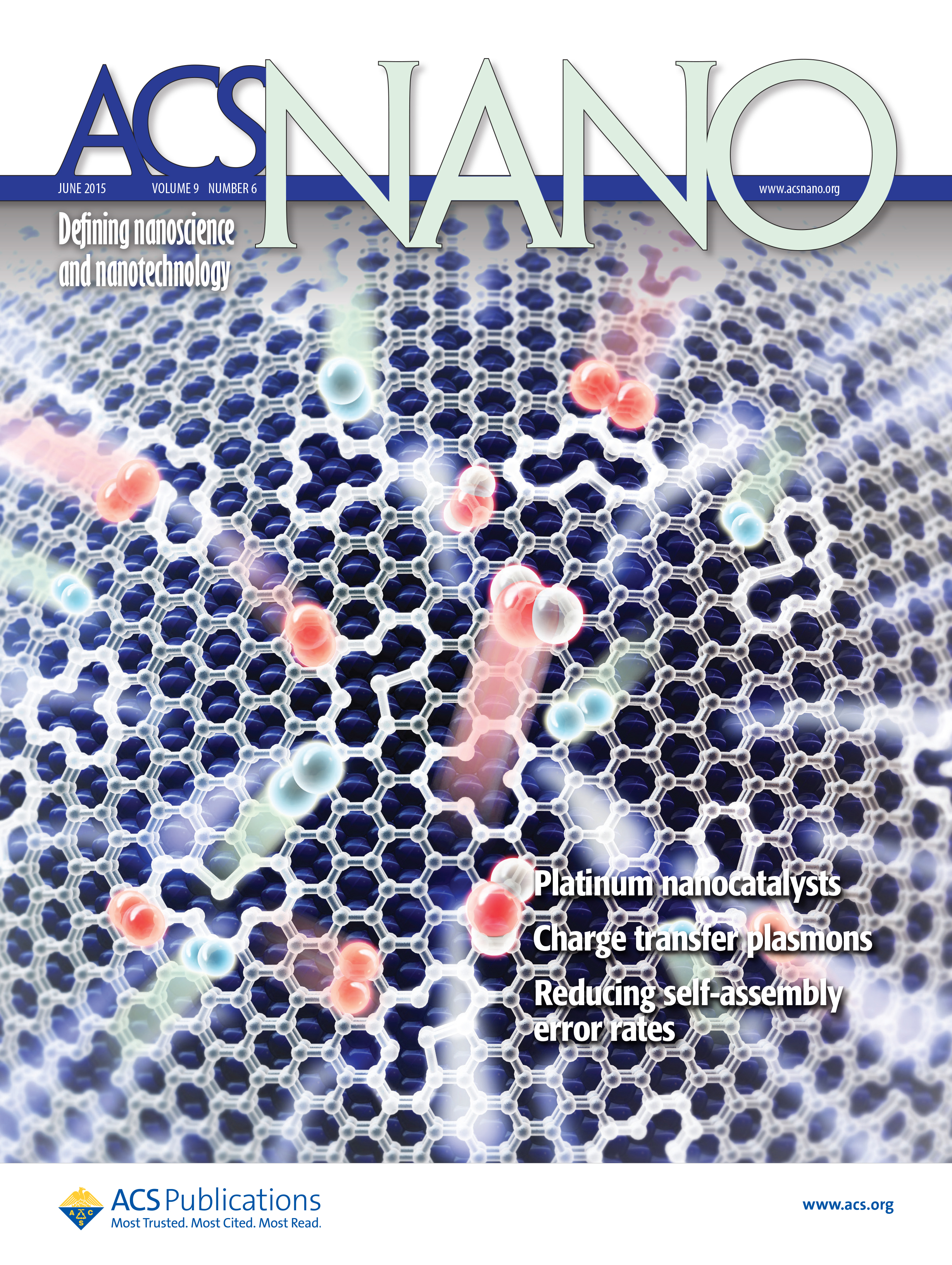
--
Capturing dendrite formation in calcium-ion batteries
ACS Energy Lett. 2020, 5, 2283-2290.Link opens in a new window
The calcium-ion rechargeable battery concept recently gained a fresh surge of interest following parallel discoveries from a coupleLink opens in a new window of groupsLink opens in a new window showing that it is possible to reversibly electrochemically cycle calcium metal, something that had been regarded as challenging prior due to its predisposition to forming an ionically 'passivating' interphase layer. Here, we performed in situ electrochemical liquid-cell TEM to capture the formation of calcium dendrites when electroplating Ca under high current densities; i.e., in the diffusion limit. We also explore some of the mechanisms by which Ca dendrites may form at lower current densities.
--
--
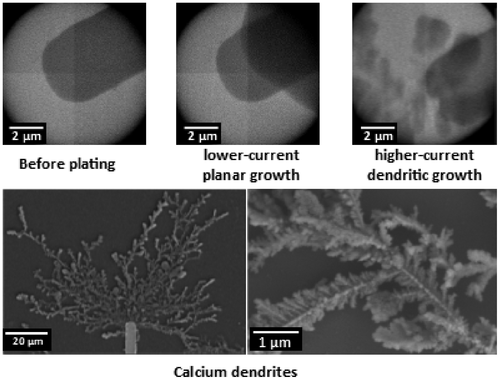
--
--

How do fundamental defects evolve at the atomic level?
Science Adv. 2020, 6, 2283-2290Link opens in a new window.
Defects, such as dislocations and dopants, are fundamental elements that determine a material's ultimate properties. How these defects change and move through the host material's lattice structure is difficult to resolve, as these processes occur at the atomic level and at high speeds. By combining our atomic resolution TEM imaging of graphene with time-resolved tight-binding molecular dynamics modelling of collaborators in Korea (Seoul National University), we are able to identify a mechanism that uses a self-interstitial "mediator" adatom, which facilitates defect evolution with a low energy barrier.
--
Epitaxial matching between graphene and atomically-thin platinum metal layers
ACS Nano. 2019, 13, 12162-12170.Link opens in a new window
Devising more efficient and robust platinum-based electrocatalysts is essential for a host of industrially important reactions, as well as for future systems that are not yet economically viable. Collaborators in the USA (Georgia Institute of Technology) had previously devised a methodLink opens in a new window to deposit atomically-thin layers of Pt onto a graphene substrate. We found that the formed platinum layers, mostly two atoms thick, had a distinct crystal structure to that of bulk Pt, and this was due to the energetics of maintaining epitaxial matching with the underlying graphene substrate. We use atomic resolution TEM imaging to reveal this, and explore the grain structure evolution of the Pt film.
--
--
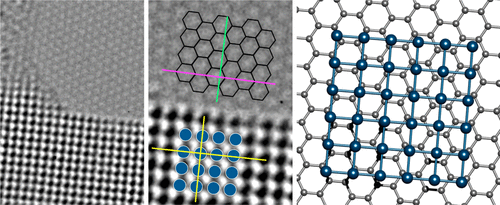
--
--
--
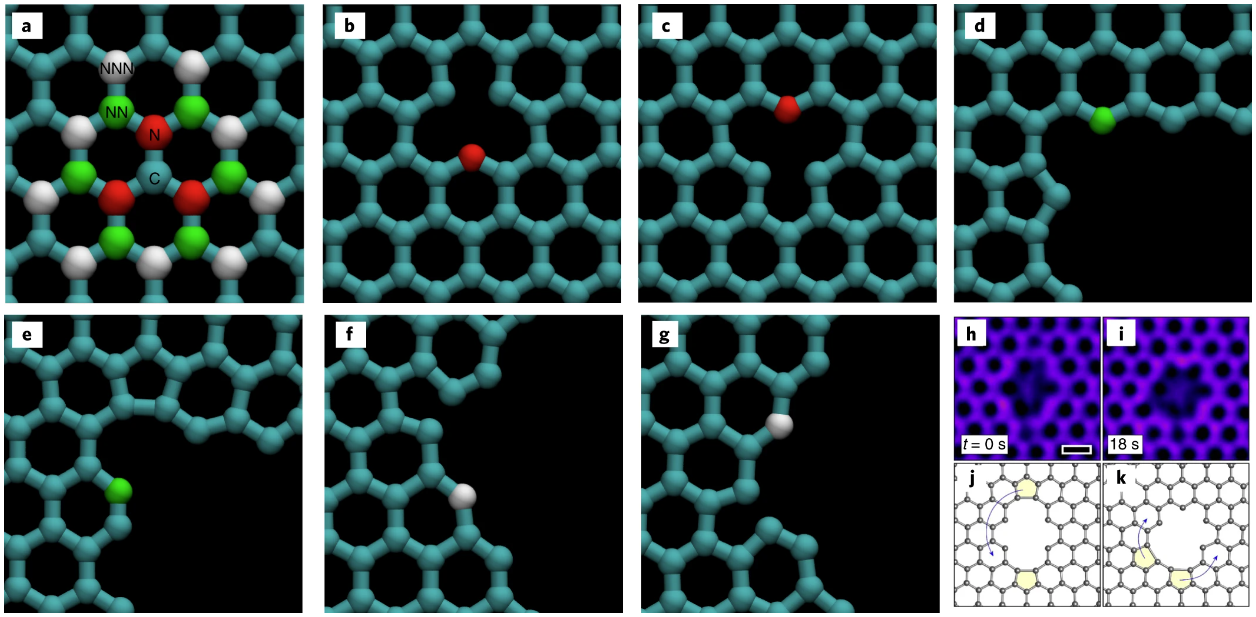
Mapping out the possibility space for different nanopore shapes in 2D materials
Nature Materials. 2019, 18, 129-135Link opens in a new window.
2D materials like graphene have been shown to make for promising molecular sieves, allowing for filtering gases, DNA, and desalination. The nanopores that decorate these membranes to allow such filtering can have a variety of atomic structures. As the pore size grows this parameter space of available atomic configurations expands exponentially. Our atomic resolution experimental TEM datasetLink opens in a new window of nanopores in graphene was used by modelling collaborators in the USA (Massachusetts Institute of Technology) as a foundation for devising a framework for describing how pores are likely to configure for a given pore size.