Quantum materials under extreme conditions
The Project
The exploration of new and exotic states of matter is as fundamental to our understanding of the universe as is the detection of elementary particles or the discovery of celestial objects. What is more, many of these states exhibit properties that could have significant impact upon future technologies.
States of particular current interest include unconventional superconductors, low-dimensional ordered magnets, spin liquids and ices, topological insulators, bosonic superfluids, shape memory phases and multiferroics. All of these examples emerge from a complex soup of many-body quantum interactions, making them difficult systems to understand. Nevertheless, finding out how the states arise is the first, but essential step towards fully harnessing their capacity for application.
This project, which ran between 2016 and 2022 and was funded by the ERC, seeks to advance our knowledge of these issues by using extreme conditions of magnetic field and pressure to enable a continuous, clean and reversible tuning of quantum interactions, thereby shedding light on the building blocks of exotic magnetism and unconventional superconductivity. The overall objective is to better understand how quantum interactions and fluctuations, topology and disorder can give rise to states of matter with novel and functional properties.
Team members
Team members who have worked on this project include: William Blackmore, Mathew Coak, Sam Curley, Paul Goddard, Katrin Götze, Matthew Pearce, Shroya Vaidya and Robert Williams.
Project publications
Below are details of some of the results that have emerged from the project.
Fermi surface transformation at the pseudogap critical point of a cuprate superconductor
Yawen Fang et al. Nature Physics 16, 558 (2022)Link opens in a new window
The nature of the pseudogap phase remains a major puzzle in our understanding of cuprate high-temperature superconductivity. Whether or not this metallic phase is defined by any of the reported broken symmetries, the topology of its Fermi surface remains a fundamental open question. In a complementary study to our 2021 Nature paperLink opens in a new window, we use angle-dependent magnetoresistance (ADMR) to measure the Fermi surface of the La1.6–xNd0.4SrxCuO4 cuprate. Outside the pseudogap phase, we fit the ADMR data and extract a Fermi surface geometry that is in excellent agreement with angle-resolved photoemission data. Within the pseudogap phase, the ADMR is qualitatively different, revealing a transformation of the Fermi surface. We can rule out changes in the quasiparticle lifetime as the sole cause of this transformation. We find that our data are most consistent with a pseudogap Fermi surface that consists of small, nodal hole pockets, thereby accounting for the drop in carrier density across the pseudogap transition found in several cuprates.
Pressure-induced shift of effective Ce valence, Fermi energy and phase boundaries in CeOs4Sb12
Kathrin Götze et al. New Journal of Physics, 24, 043044 (2022)Link opens in a new window
In a follow-up to our 2020 PRB paperLink opens in a new window on the same material, we have conducted measurements of electrical transport and megahertz conductivityon CeOs4Sb12 single crystals under pressures of up to 3 GPa and in high magnetic fields of up to 41 T to investigate the influence of pressure on the different H–T phase boundaries. While the high-temperature valence transition between the metallic H-phase and the L-phase is shifted to higher T by pressures of the order of 1 GPa, we observed only a marginal suppression of the S-phase that is found below 1 K for pressures of up to 1.91 GPa. High-field quantum oscillations have been observed for pressures up to 3.0 GPa and the Fermi surface of the high-field side of the H-phase is found to show a surprising decrease in size with increasing pressure, implying a change in electronic structure rather than a mere contraction of lattice parameters. We evaluate the field-dependence of the effective masses for different pressures and also reflect on the sample dependence of some of the properties of CeOs4Sb12 which appears to be limited to the low-field region.
Magnetic monopole density and antiferromagnetic domain control in spin-ice iridates
Matthew J. Pearce et al. Nature Communications 13, 444 (2022).
Magnetically frustrated systems provide fertile ground for complex behaviour, including unconventional ground states with emergent symmetries, topological properties, and exotic excitations. A canonical example is the emergence of magnetic-charge-carrying quasiparticles in spin-ice compounds. Despite extensive work, a reliable experimental indicator of the density of these magnetic monopoles is yet to be found.
Using measurements on single crystals of Ho2Ir2O7 combined with dipolar Monte Carlo simulations, we show that the isothermal magnetoresistance is highly sensitive to the monopole density. Moreover, we uncover an unexpected and strong coupling between the monopoles on the holmium sublattice and the antiferromagnetically ordered iridium ions. These results pave the way towards a quantitative experimental measure of monopole density and demonstrate the ability to control antiferromagnetic domain walls using a uniform external magnetic field, a key goal in the design of next-generation spintronic devices.
The figure is a cartoon showing the effect of a [111] applied magnetic field on the density of magnetic monopoles (circles), leading to the preferential growth of a particular antiferromagnetic domain
Magneto-structural Correlations in Ni2+—Halide···Halide—Ni2+ Chains
William J. A. Blackmore et al. Inorg. Chem. 61, 141 (2022).Link opens in a new window
This study presents the magnetic properties of a new family of S = 1 molecule-based magnets, NiF2(3,5-lut)4·2H2O and NiX2(3,5-lut)4, where X = HF2, Cl, Br, or I (lut = lutidine C7H9N). Upon creation of isolated Ni–X···X–Ni and Ni–F–H–F···F–H–F–Ni chains separated by bulky and nonbridging lutidine ligands, the effect that halogen substitution has on the magnetic properties of transition-metal-ion complexes can be investigated directly and in isolation from competing processes such as Jahn–Teller distortions. We find that substitution of the larger halide ions turns on increasingly strong antiferromagnetic interactions between adjacent Ni2+ ions via a novel through-space two-halide exchange. In this process, the X···X bond lengths in the Br and I materials are more than double the van der Waals radius of X yet can still mediate significant magnetic interactions. We also find that a simple model based on elongation/compression of the Ni2+ octahedra cannot explain the observed single-ion anisotropy in mixed-ligand compounds. We offer an alternative that takes into account the difference in the electronegativity of axial and equatorial ligands.
Anomalous magnetic exchange in a dimerized quantum magnet composed of unlike spin species
Samuel P. M. Curley et al. Phys. Rev. B 104, 214435 (2021).Link opens in a new window
Here, we present the magnetic properties of the antiferromagnetic dimer material CuVOF4(H2O)6⋅H2O, in which the dimer unit is composed of two different S=1/2 species, Cu(II) and V(IV). An applied magnetic field of 13.1(1) T is found to close the singlet-triplet energy gap, the magnitude of which is governed by the antiferromagnetic intradimer J0 ≈ 21 K and interdimer J′ ≈ 1 K exchange energies, determined from magnetometry and electron-spin resonance measurements. The results of density functional theory (DFT) calculations are consistent with the experimental results. The DFT calculations predict antiferromagnetic coupling along all nearest-neighbor bonds, with the magnetic ground state comprising spins of different species aligning antiparallel to one another, while spins of the same species are aligned parallel. The magnetism in this system cannot be accurately described by the overlap between localized V orbitals and magnetic Cu orbitals lying in the Jahn-Teller (JT) plane, with a tight-binding model based on such a set of orbitals incorrectly predicting that interdimer exchange should be dominant. DFT calculations indicate significant spin density on the bridging oxide, suggesting instead an unusual mechanism in which intradimer exchange is mediated through the O atom on the Cu(II) JT axis.
Linear-in temperature resistivity from an isotropic Planckian scattering rate
Gaël Grissonnanche et al. Nature 595, 667 (2021).Link opens in a new window
The Planckian limit is believed to be the upper boundary on scattering of charge carriers in a conducting system, and has been attributed as the origin of the linear-in-temperature resistivity observed in several 'strange metals' in recent years. In this limit, the scattering rate is predicted to depend only on fundamental constants and the temperature.
Here, we (a collaborative team from Cornell, Sherbrooke, Paris-Saclay, Texas, Warwick, and the National High Magnetic Field Laboratory) made use of angle-dependent magnetoresistance, a technique in which electronic transport properties are monitored while rotating the sample in a large magnetic field. The team found that the results measured in a high-temperature cuprate superconductor could be readily explained with a surprisingly simple transport model including an isotropic scattering rate that is right at the predicted Planckian limit.
The key to the result lies in the careful analysis of the data, which sheds new light on this fundamental limit for electronic motion. The findings also underline the benefit of the angle-dependent magnetoresistance technique, which provides essential information that is very difficult to uncover in any other way.
Magnetic ground state of the one-dimensional ferromagnetic chain compounds M(NCS)2(thiourea)2 (M = Ni, Co)
Samuel P. M. Curley et al. Physical Review Materials 5, 034401 (2021)Link opens in a new window
Here the magnetic properties of the two isostructural molecule-based magnets—Ni(NCS)2(thiourea)2, S=1 [thiourea=SC(NH2)2] and Co(NCS)2(thiourea)2, S=3/2—are characterized using several techniques in order to rationalize their relationship with structural parameters and to ascertain magnetic changes caused by substitution of the spin. Electron charge- and spin-density mapping reveals through-space exchange as a mechanism to explain the large discrepancy in magnetic interactions despite, from a structural perspective, the highly similar exchange pathways in both materials.
Controlling Magnetic Anisotropy in a Zero-Dimensional S = 1 Magnet Using Isotropic Cation Substitution
J. L. Manson et al. J. Am. Chem. Soc. 143, 4633 (2021)Link opens in a new window
Here we describe a novel form of symmetry breaking driven by chemical intervention: an anisotropic change in magnetic properties is induced by ionic substitution of an isotropic species.
The chemical system in question is a three-dimensional hybrid organic-inorganic framework with different molecular bridges linking spin-1 Ni(II) ions along the axial and equatorial directions. The study makes use of a raft of complementary techniques that includes x-ray diffraction, magnetometry, susceptometry, x-ray fluorescence and ESR, backed up by data modelling and percolation calculations. We find that replacing 80% of the anisotropic Ni(II) with isotropic Zn(II) ions induces a 22% enhancement in single-ion anisotropy. This substantial change is accomplished by an anisotropic lattice expansion and exploits the relative donor atom hardness as a structural design tool.
In this way it is possible to tune the single-ion anisotropy of a magnetic lattice site by Zn-substitution on nearby sites, suggesting that the combination of anisotropic organic frameworks and metal ions of different radii may be exploited to tune the single-ion properties of magnetically isolated spins to desired values. This will be useful in the control of magnetization reversal and slow relaxation in self-assembled arrays of single-ion magnets composed of d− or f−elements for use in spin-based electronic devices.
Magnetic order and ballistic spin transport in a sine-Gordon spin chain
Physical Review B 103, L060405 (2021)
This study follows on from our discovery of a chiral staggered S = 1/2 chain (see below and here). In this letter, via collaboration with muon-spin spectroscopists from Durham, Oxford and the ISIS Neutron and Muon Facility, we investigate the spin transport in the non-chiral staggered chain [pym-Cu(NO3)2(H2O)2] (pym = pyrimidine), whose low-temperature properties are well described by the sine-Gordon field theory. Long-range magnetic ordering is found in this material for the first time below 230 mK, as well as evidence for ballistic spin transport. This is in contrast to the chiral chain [Cu(pym)(H2O)4]SiF6⋅H2O for which no magnetic order is seen down to the lowest temperatures and diffusive spin transport is detected. The distinct spin transport in these two systems suggests that anisotropic interactions (such as Dzyaloshinskii-Moriya) play an important role in determining the nature of spin transport in S = 1/2 antiferromagnetic chains.
Magnetic order and disorder in a quasi-two-dimensional quantum Heisenberg antiferromagnet with randomized exchange
Physical Review B 102, 174429 (2020)
We investigated the effect of randomizing exchange coupling strengths in the S = 1/2 square lattice quasi-two-dimensional quantum Heisenberg antiferromagnet (QHAF) (QuinH)2Cu(ClxBr1−x)4⋅2H2O (QuinH = Quinolinium, C9H8N+), with 0 ≤ x ≤ 1. The figure on the left shows a simulation of the two-dimensional lattice in for different values of x. The colouring of the bonds is black for Br–Br, yellow for Br–Cl, red for Cl–Cl.
Pulsed-field magnetization measurements allow us to estimate an effective in-plane exchange strength J in a regime where exchange fosters short-range order, while the temperature TN at which long-range order (LRO) occurs is found using muon-spin relaxation, allowing us to construct a phase diagram for the series. We evaluate the effectiveness of disorder in suppressing TN and the ordered moment size, and we find an extended disordered phase in the region 0.4 ≲ x ≲ 0.8 where no magnetic order occurs. The observed critical substitution levels are accounted for by an energetics-based competition between different local magnetic orders. Furthermore, we demonstrate experimentally that the ground-state disorder is driven by quantum effects of the exchange randomness, which is a feature that has been predicted theoretically and has implications for other disordered quasi-two-dimensional QHAFs.
Extremely well isolated two-dimensional spin-1/2 antiferromagnetic Heisenberg layers with a small exchange coupling in the molecular-based magnet CuPOF
Physical Review B 102, 064431 (2020)
We report on a comprehensive characterization of the newly synthesized Cu(II)-based molecular magnet. From a comparison of theoretical modeling to results of bulk magnetometry, specific heat, μSR, ESR, and NMR spectroscopy, this material is determined as an excellent realization of the two dimensional square-lattice S = 1/2 antiferromagnetic Heisenberg model with a moderate intraplane nearest-neighbor exchange coupling of J/kB = 6.80(5) K, and an extremely small interlayer interaction of about 1 mK. At zero field, the bulk magnetometry reveals a temperature-driven crossover of spin correlations from isotropic to XY type, caused by the presence of a weak intrinsic easy-plane anisotropy. A transition to long-range order, driven by the low-temperature XY anisotropy under the influence of the interlayer coupling, occurs at TN =1.38(2) K, as revealed by μSR. In applied magnetic fields, our 1H-NMR data reveal a strong increase of the magnetic anisotropy, manifested by a pronounced enhancement of the transition temperature to commensurate long-range order at TN = 2.8 K and 7 T.
Enhancing easy-plane anisotropy in bespoke Ni(II) quantum magnets
We examine the crystal structures and magnetic properties of several S = 1 Ni(II) coordination compounds, molecules and polymers. Pseudo-octahedral NiN4F2, NiN4O2 or NiN4OF cores consist of equatorial Ni-N bonds that are equal to or slightly longer than the axial Ni-Lax bonds. By design, the zero-field splitting (D) is large in these systems and, in the presence of substantial exchange interactions (J), can be difficult to discriminate from magnetometry measurements on powder samples. We use pulsed-field magnetization in those cases and employ electron-spin resonance to confirm D when J ≪ D. The anisotropy of each compound was found to be easy-plane (D > 0) and range from ≈ 8–25 K. This work reveals a linear correlation between the ratio d(Ni-Lax)/d(Ni-Neq) and D, although the ligand spectrochemical properties are expected also to play an important role. This relationship allows us to predict the type of magnetocrystalline anisotropy in tailored Ni(II) quantum magnets.
Unusual phase boundary of the magnetic-field-tuned valence transition in CeOs4Sb12
Physical Review B 101, 075102 (2020)Link opens in a new window
Here we map the temperature–magnetic field phase diagram of the filled skutterudite material CeOs4Sb12 in fields up to 60 T and temperatures down to 0.5 K.
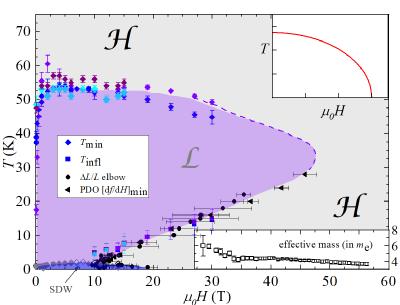
We find that the valence transition separating the semimetallic low-field, low-temperature ‘L’ phase from the metallic high- field, high-temperature ‘H’ phase exhibits a very unusual, wedge-shaped phase boundary, contrary to expectations from thermodynamic constraints which predict an elliptical phase boundary. The low-temperature, high-field behaviour of the phase boundary is attributed to magnetic fluctuations associated with a quantum critical point identified by a marked enhancement of the electronic effective mass. In addition, a strong sample dependence of the high-temperature, low-field side of the valence transition is ascribed to CeOs4Sb12 being on the verge of becoming a topological semimetal triggered by uniaxial strain
Valence transitions, driven by magnetic field, pressure and/or temperature are a highly and widely debated topic in the condensed-matter community. The phase diagrams of a number of important systems, such as Pu and URu2Si2, are discussed in the context of valence transitions and their underlying energy scales. Field-induced valence transitions usually follow an elliptical phase boundary for which H2 is proportional to T2. CeOs4Sb12, a close relative of the unconventional superconductor PrOs4Sb12 and the ferromagnetic NdOs4Sb12, deviates significantly from this universal behaviour and shows a strongly altered boundary of its field-tuned valence transition. This alteration of a so far well-understood phase boundary will advance the understanding of valence transitions tuned by external parameters and stimulate further discussion on valence transitions affected by quantum criticality and topology.
SquidLab – a user-friendly program for background subtraction and fitting of magnetization data
Review of Scientific Instruments 91, 023901 (2020)
Measurements of magnetic properties in the lab are commonplace and essential across a range of physics, chemistry, materials science and even biology. There are many cases however where the magnetic signal of the sample holder or environment swamps that of the material to measure. Our new software, SquidLab, provides a powerful and flexible toolbox to remove magnetic backgrounds to recover the sample's signal and allow measurements that were previously impossible.
The user-friendly programme is written and run in MATLAB and can be customised and integrated with the user's own processing algorithms. After the user uploads a sample and a background dataset, SquidLab conducts multidimensional interpolation to ensure the data are at the same external conditions, such as magnetic field and temperature. It then performs the background subtraction and fits the data to the expected magnetic dipole form in a variety of customisable methods. The original use case was for high-pressure studies, but SquidLab is quickly proving an invaluable tool for a wide host of applications in magnetic measurement.
Near-ideal molecule-based Haldane spin chain
Physical Review Research 2, 013082 (2020)
It is well known that quantum interactions work on a very small scale. Nevertheless, the phenomena and physical properties that emerge from these interactions are fascinating and potentially useful.
Making materials, especially magnetic materials, that accentuate quantum effects is an active area of research and it is now well established that an effective avenue is to restrict the interactions to just one or two dimensions. Particularly interesting are systems of interacting magnetic particles arranged in chains. Theoretical physicist Duncan Haldane famously predicted that depending on the precise quantum mechanical details of the individual particles, all such chains should fall into one of two types distinguished by wildly different macroscopic properties. For this he shared the 2016 Nobel Prize in Physics.
A hinderance to progress in this area is that while theoretical models of magnetic chains can be constructed relatively easily, making real materials corresponding to these models has proved to be very difficult, stymying experimental testing of quantum theories. Our paper describes how we make use of recent breakthroughs in the field of metal-organic hybrid materials to build a highly one-dimensional magnetic system and, by careful characterization using multiple experimental techniques, we show that the new material is a uniquely ideal real example of a Haldane chain.
An added advantage is that the magnetic interactions in our material can be tuned using accessible magnetic fields. This opens up the type of measurements that can be performed and will make possible a deeper understanding of the fundamentals of quantum magnetism.
Determining the anisotropy and exchange parameters of polycrystalline spin-1 magnets
New Journal of Physics 21, 093025 (2019)
While experiments should ideally be performed on single-crystals where possible, it is clearly advantageous to be able to characterise the basic properties of a polycrystalline anisotropic magnetic material using simple, readily accessible measurement techniques in order to identify the compounds that merit the often considerable additional work required for high-quality crystal growth. However, the complication of powder-averaging leads to difficulties interpreting the results of bulk thermodynamic measurements.
Here we show how to overcome these difficulties. We test the efficacy of our method using three new magnetic materials and show that we are able to extract the anisotropy parameters in each case. We also present a density functional method, which incorporates spin–orbit coupling to estimate the size of the anisotropy in S = 1 magnets.
Unconventional Field-Induced Spin Gap in an S = 1/2 Chiral Staggered Chain
Physical Review Letters 122, 057207 (2019)
We have measured the quantum spin chain [Cu(pyrimidine)(H2O)4]SiF6.H2O, which has a chiral structure and intriguing properties.
Typically the theory of low-dimensional magnetism precedes experimental testing, largely because of the difficulties in creating real materials described by theoretical models. However, in some cases experimental work uncovers physics not previously predicted. An example is the spin chain copper-benzoate where the local environment around the spins alternates along the chain. In this case, experiments revealed that a magnetic energy gap forms when a magnetic field is applied.
This was entirely perplexing until Oshikawa and Affleck found that the behaviour could be described by the sine-Gordon model of quantum-field theory. Their theoretical work inspired a great deal more activity.
In this paper, we highlight a new material, which at first glance could be a sine-Gordon chain, but with an added twist: a four-fold chiral structure. Using heat capacity, magnetometry and electron-spin resonance measurements, we show that the size and field-dependence of the energy gap, as well as the complex excitation spectrum, do not fit with the existing theories. We offer a qualitative explanation for the observations, however considerable theoretical effort is called for to quantitatively account for the results. The study also raises the possibility of combining different chiral symmetries with anisotropic interactions to create new ground states and exotic excitations.
Magnetic order and enhanced exchange in the quasi-one-dimensional molecule-based antiferromagnet Cu(NO3)2(pyrazine)3
Phys. Chem. Chem. Phys. 21, 1014 (2019)
An ongoing research effort is to create extreme one-dimensional spin-1/2 chains to accentuate the effect of quantum fluctuations. In January 2019 we published the paper linked above. Here we discuss the quasi-one-dimensional molecule-based Heisenberg antiferromagnet Cu(NO3)2(pyz)3, which has an intrachain coupling J = 13.7(1) K and exhibits a state of long-range magnetic order below T = 0.105(1) K. The ratio of interchain to intrachain coupling is estimated to be |J′/J| = 3.3 × 10−3, demonstrating a high degree of isolation for the Cu chains. In the paper we discuss the possible reasons why the chains in this system appear to be more isolated than in similar molecule-based materials.
Implications of bond disorder in a S=1 kagome lattice
Scientific Reports 8, 4745 (2018)
Long-range magnetic order (LRO) in low-dimensional materials is often the result of a delicate balance of competing interactions. This balance can be perturbed in a number of ways. Typically, the effect of introducing random disorder into a system of interacting spins is to suppress the onset of an ordered state.
However, the inherent structural configuration of some magnetic lattices (e.g. triangular, hexagonal, kagome) gives rise to a geometric frustration of the dominant exchange interactions, which itself precludes LRO, leading to a large ground-state degeneracy and the possibility of spin-liquid behaviour. In general, introducing structural disorder into such a system will act to lift the frustration and restore LRO.
The complex interplay between these three phenomena (order, disorder and frustration) is an area of considerable current interest. In this paper we set out to synthesize an ideally frustrated kagome lattice of S = 1 spins in a tunable molecule-based crystal. Unexpectedly, the material that self-assembles exhibits an unusual randomized network in which one-in-three magnetic exchange pathways is slightly distorted. This random structural disorder lifts the effect of magnetic frustration and should promote the onset of LRO. However, the surprising additional consequence is that the small distortion acts to suppress the size of the effective exchange energies, impeding LRO down to milli-Kelvin temperatures. Only with a combination of detailed magnetic investigations and density-functional theory were we able to understand the properties of this material.
Combining microscopic and macroscopic probes to untangle the single-ion anisotropy and exchange energies in an S = 1 quantum antiferromagnet
Physical Review B, 95, 134435 (2017)
Many of the newest and most exciting materials are frequently only available in powdered form. This can lead to difficulties in determining the energy scales and anisotropy of competing interactions, particularly when these energies are similar in magnitude.
For this paper, we synthesized a quasi-1D molecular antiferromagnet, [Ni(HF2)(pyrazine)2]SbF6, composed of Ni(II) moments and H···F hydrogen bridges. Further connectivity through pyrazine ligands affords a robust 3D structural network. Such S = 1 chains are of interest especially if the single-ion anisotropy D and magnetic exchange energy J can be controlled and tuned. We show that a combination of high magnetic fields and neutron scattering could reveal and untangle these parameters, an unprecedented feat in a powder considering D/J is approximately 1 for this material.
While D/J is close to the experimentally elusive quantum tricritical point where Haldane, XY and quantum paramagnetic phases coexist, the small interchain exchange energy is still sufficient to prompt long-range XY antiferromagnetic order near 12 K. This work can enable characterisation of polycrystalline samples of other low-dimensional magnets with competing energy scales.
Future work involves tuning of the parameters of this material through metal-ion doping and high pressure in an effort to tip the delicate balance of interactions, pushing towards the nearby phase boundaries and into Haldane physics territory.
Adiabatic physics of a magnetic quantum fluid: magnetocaloric effect, zero-point fluctuations, and two-dimensional universal behavior
Physical Review B 95, 024404 (2017)
A key goal of physicists, materials scientists and chemists is to develop materials to test the theoretical predictions of quantum mechanics in real systems. High impact research in this field includes a focus on magnetic quantum fluids that exhibit the Bose-Einstein condensation (BEC) of magnons.
Often the experimental investigations of these systems are reliant on the results of measurements in pulsed magnetic fields. One of the results of our work is that, without careful consideration of the sample temperature in these measurements, a magnetocaloric effect can arise undetected and a naïve interpretation of the resulting data can lead to erroneous physics. This conclusion has possible implications for several existing and ongoing studies in this area, where we suggest similar distortions of the phase diagram occur.
Our results derive from the application of several state-of-the-art experimental techniques, including measurements of the susceptibility (at radio frequencies) and the magnetocaloric effect in pulsed magnets. Given these experiments revealed new physics in our material, we hope the work will encourage others to consider the consequences of studying quantum materials with ultra-high magnetic fields. Our experimental results are explained with a simple and general theoretical model and is relevant to the wider investigation of spin-gapped and correlated materials. Furthermore, we show that quantum fluctuations play a role in determining the magnetic properties of our low-dimensional system and that the critical exponents on either side of the BEC phase may be different, the possibility of which is an ongoing debate in this field.
This project has received funding from the European Research Council (ERC) under the European Union’s Horizon 2020 research and innovation programme (grant agreement No 681260).