Research Highlight: 3D surface convection in white dwarfs
White dwarfs represent the endpoint of stellar evolution for 95% of all stars. At the present day in our Galaxy, the large majority of stars that were born slightly more massive than the Sun are in their final remnant stage. These degenerate stars are slowly cooling as they lose their internal energy through radiation. We study them both for the purpose of understanding these condensed matter laboratories, and for enhancing their use as probes of fundamental astrophysical relations, such as the expansion of the Universe. The study of white dwarfs in clusters, routinely done by HST, provides very precise ages for the first stellar populations in our Galaxy. By linking the final white dwarf mass to the initial mass of its progenitor, it is also possible to calibrate the core mass growth and stellar lifetime of asymptotic giant branch (AGB) stars [1].
Most of the mass in a C/O white dwarf is a mixture of carbon and oxygen, and there is usually a thin layer of hydrogen (less than 0.01% of the mass) floating at the surface. As a consequence, most degenerate stars have a pure-hydrogen atmosphere. The most accurate method to determine the atmospheric parameters (the effective temperature and surface gravity) of H-rich white dwarfs is to compare the observed line profiles of the hydrogen Balmer lines with the predictions of detailed model atmospheres (Figure 1) [2]. Nevertheless, there was a long-standing problem [3] where cool remnants (0.2 < Cooling Age [Gyr] < 10) with a convective atmosphere have masses up to 20% higher than warmer non-convective objects, which impacts the use of white dwarfs as cosmochronometers.
Figure 1: Observed spectra of the white dwarf WD 1053−290 with a simultaneous fit of the Balmer lines, from Hβ to H8, with a 3D model spectrum. Line profiles are offset vertically from each other for clarity and the best-fit atmospheric parameters are identified at the bottom of the panels. The instrumental resolution is of 6 Å. Source: Tremblay et al. (2013b)
We have recently computed the first grid of 3D model atmospheres [4] for hydrogen-atmosphere white dwarfs (Figure 2) in order to improve the convection model. These CO5BOLD [5] radiation-hydrodynamics simulations, unlike the previous 1D calculations, do not rely on the mixing-length theory or any free parameter for the treatment of convective energy transfer.
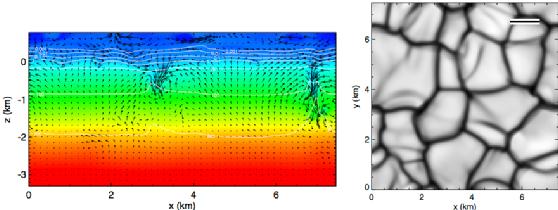
The 3D simulations have been employed to compute 3D spectra for the Balmer lines which were then used in the spectroscopic analysis of the white dwarfs in the Sloan Digital Sky Survey [6]. White dwarfs with radiative and convective atmospheres have derived mean masses that are now the same (Figure 3), in much better agreement with our understanding of stellar evolution. Indeed, both cool and warm degenerates in the Galactic disk are expected to originate from the same populations, but from stars that have formed at slightly different times. We are now in the process of using the 3D simulations as upper boundary conditions for structure models, in order to predict improved ages and more precise ZZ Ceti pulsation properties. We will also improve the metal abundance determinations for white dwarfs that are accreting former disrupted planets in their convective zone.
Figure 3: Mass histograms for DA stars in the Sloan Digital Sky Survey sample with Teff < 40 000 K (black empty histogram) from 1D (top) and 3D spectra (bottom). We also show the sub-distributions for radiative atmospheres (13 000 < Teff (K) < 40 000, blue histogram) and convective atmospheres (Teff < 13 000 K, red histogram). The mean masses and standard deviations are indicated in the panels in units of solar masses. Binaries and magnetic objects were removed from the distributions. Source: Tremblay et al. (2013b)
References:
[1] Kalirai, J. S., Marigo, P., & Tremblay, P.-E. 2014 (ApJ, 782, 17)
[2] Bergeron, P., Saffer, R. A., & Liebert, J. 1992 (ApJ, 394, 228)
[3] Bergeron, P., Wesemael, F., Fontaine, G., & Liebert, J. 1990 (ApJL, 351, L21)
[4] Tremblay, P.-E., Ludwig, H.-G., Steffen, M., & Freytag, B. 2013a (A&A, 552, A13)
[5] Freytag, B., Steffen, M., Ludwig, H.-G., et al. 2012 (Journal of Computational Physics, 231, 919)
[6] Tremblay, P.-E., Ludwig, H.-G., Steffen, M., & Freytag, B. 2013b (A&A, 559, A104)